Integrating Parasitoid Olfactory Conditioning in Augmentative Biological Control: Potential Impact, Possibilities, and Challenges
- 1Wageningen University and Research, Greenhouse Horticulture, Bleiswijk, Netherlands
- 2Department of Terrestrial Ecology, Netherlands Institute of Ecology (NIOO-KNAW), Wageningen, Netherlands
- 3Laboratory of Entomology, Wageningen University and Research, Wageningen, Netherlands
Despite the vast body of theoretical and empirical literature dealing with parasitoid learning, this knowledge has thus far rarely been exploited for manipulating the efficacy of augmentative biological pest control. This may be due to the fact that most studies on learning behavior were performed under laboratory conditions, whereas field trials remain scarce. However, the few studies that did investigate parasitoid foraging success under (semi-)field conditions show strong learning effects. Using so-called “parasitoid olfactory conditioning” (POC), parasitoids can be trained to become more efficient in the different phases involved in the process of host searching and host acceptance. POC can thus result in a “foraging efficacy gain”, defined as the difference between the number of naive and conditioned parasitoids that need to be released to reach a certain parasitization level of the target-pest in the crop environment. This “gain” increases with an improved parasitoid learning ability and depends on the interplay between the parasitoid, crop, target-pest species and parasitoid rearing method. Moreover, the “foraging efficacy gain” depends on the technical implementation of POC, as this will determine the strength, duration and stability of the learning-induced behavioral change. In this perspective paper we will discuss (a) the conditions that can enhance the “foraging efficacy gain,” (b) the possible approaches to implementation of POC and their costs and benefits, and (c) a stepwise approach to develop appropriate POC methods for the optimization of biological pest control.
Introduction
Augmentative biocontrol using predatory arthropods or parasitoid wasps has become a major way to reduce insecticide use, especially in protected horticulture (Pilkington et al., 2010; van Lenteren et al., 2018). The efficacy of parasitoids to find and eliminate pest insects, however, may be constrained by an initially weak response to foraging cues emanating from the crop environment, and/or an innate tendency for dispersal upon release. Learning can greatly enhance the efficacy of parasitoids to locate hosts under field or greenhouse conditions (Gross et al., 1975; Lewis and Martin, 1990; Papaj and Vet, 1990; Hare et al., 1997; White and Andow, 2007; Wilson and Woods, 2016). Using so-called “parasitoid olfactory conditioning” (POC from here on; Box 1) parasitoids can be trained to enhance their searching activity, their ability to locate target-pest infested plants and target-pest insects, as well as their acceptance of the target-pest for egg-laying. The aims of this perspective paper are to discuss (a) the conditions under which the impact of POC on biological pest control will be highest, (b) the costs and benefits of possible approaches to POC implementation, and (c) a stepwise approach for the development of POC methods for the optimization of biological pest control. For a detailed overview of current knowledge on parasitoid learning and memory we refer to the recent reviews of Hoedjes et al. (2011), Giunti et al. (2015), Smid and Vet (2016), and Nieberding et al. (2018).
Box 1. Definition of terms used in this paper.
Parasitoid olfactory conditioning (POC): defined here as training parasitoids to respond more strongly and/or specifically to odors involved in target-pest-finding or target-pest-acceptance by making use of one or more of the learning mechanisms outlined below.
Associative learning: involves the establishment, through experience, of an association between two stimuli (classical conditioning) or between a stimulus and a response (operant conditioning). Typically, the association is formed through the close temporal and spatial pairing of the stimuli or the stimulus and response (Papaj and Prokopy, 1989). In the context of this paper, target pest-derived odors serve as rewards in the associative learning of better detectable, but less reliable target-pest induced crop volatiles.
Sensitization: A form of non-associative learning, characterized by a gradual increase in response to a stimulus with (repeated) exposure to that stimulus even when it has not been paired with any other stimulus. Sensitization is often considered as the counterpart of habituation (Papaj and Prokopy, 1989).
Priming: A form of non-associative learning characterized by a general increase in responsiveness to foraging cues after a certain experience (Turlings et al., 1993). Conversely, associative learning, sensitization and habituation are characterized by a change in responsiveness to specific stimuli that the parasitoid encounters during the experience.
NB: It is important to note that the different types of learning outlined above are not necessarily independent of one another (e.g., mechanisms of sensitization can be involved in associative learning and any learning procedure is likely to involve priming).
Foraging efficacy gain: defined here as the difference between the number of naive and conditioned parasitoids that need to be released to reach a certain parasitization level of the target-pest in the crop environment.
Conditioned parasitoid: defined here as a parasitoid that has had experience with odors that are characteristic for the target-pest or for the crop in which it has been released. This experience could have been gained during its development (pre-adult learning), during eclosion from the pupal case (early-adult learning) or during the adult stage (adult learning).
Naive parasitoid: defined here as a parasitoid that during its development, during eclosion from the pupal case or during the adult stage has had no experience with odors that are characteristic for the target-pest or for the crop in which it has been released.
The Role of Learning in Parasitoid Foraging Behavior
Parasitoids need to complete three host-searching phases, namely (a) the initiation of host-searching behavior, (b) the detection of a host-infested plant, and (c) the detection and acceptance of the host itself (Vinson, 1976). Because pests have generally evolved toward becoming as inconspicuous as possible to their natural enemies, parasitoids usually need to resort to indirect cues, such as herbivore-induced plant volatiles (HIPVs) (Vet and Dicke, 1992; McCormick et al., 2012; Wajnberg and Colazza, 2013). HIPVs, which are emitted by the plant in response to herbivore feeding, are generally better detectable than host-derived stimuli but are less reliable as predictors of host presence. Parasitoids can use associative learning (Box 1) to solve this so-called “reliability-detectability” dilemma, by associating the less reliable HIPVs to host presence (Vet et al., 1991). This can cause parasitoids to increase their response level toward the learned HIPVs, possibly resulting in a preference shift and temporary specialization. Moreover, associative learning of HIPVs can cause parasitoids to move in a more directed manner toward a target-pest infested plant (Vet and Groenewold, 1990; Vet and Papaj, 1992; Ishii and Shimada, 2010). Apart from “associative learning,” also other learning processes, including, “sensitization,” “habituation” and “priming” may play a role in the host location process (Box 1). Moreover, parasitoid learning may take place at different stages of parasitoid ontogenesis. Here, we distinguish between “adult learning,” “early adult learning” and “pre-adult learning” (see the definition of “conditioned parasitoid” in Box 1).
Effect of POC on Parasitoid Host Searching Efficacy in the Field
Despite overwhelming laboratory-based evidence that parasitoids can learn, few attempts have been made to demonstrate the benefit of learning for optimizing host searching efficacy under (semi)field conditions (but see Gross et al., 1975; Lewis and Martin, 1990; Papaj and Vet, 1990; Hare et al., 1997; White and Andow, 2007; Wilson and Woods, 2016; Kruidhof et al., unpublished results). Papaj and Vet (1990) showed that Leptopilina heterotoma females that had experienced the host microhabitat odor in the presence of Drosophila host larvae had a 3–4 times higher chance of finding a host microhabitat than inexperienced females. Moreover, Kruidhof et al. (unpublished results) found Cotesia glomerata females released in a semi-natural environment one day after associative POC to have a twice as high host finding rate compared to inexperienced females. Other studies have found that the early adult exposure or pre-release exposure of parasitoid females to host-related stimuli (such as feces, scales, or synthetic kairomones) in the absence of the host microhabitat odor can also enhance host finding and parasitization efficacy under semi-field conditions (Gross et al., 1975; Lewis and Martin, 1990; Hare and Morgan, 1997). The results of Gross et al. (1975) suggest that parasitoids with a strong innate tendency to disperse away from the crop environment without initiating host-searching behavior may be more affected by conditioning compared to parasitoids that disperse at short distances to search for hosts.
The Potential Impact of Parasitoid Conditioning Approaches on Parasitoid Foraging Efficacy
To facilitate thinking about the conditions under which parasitoid training through POC may provide a benefit for biological pest control, we first introduce the term “foraging efficacy gain,” defined as the difference between the number of naive and conditioned parasitoids that need to be released to reach a certain parasitization level of the target-pest in the crop environment. This “gain” increases with an improved parasitoid learning ability and depends on the interplay between the parasitoid, crop, target-pest species, and parasitoid rearing method. This can be depicted as a tetrahedron, with each side of this tetrahedron corresponding to a different stage in the host searching process (Figure 1A).
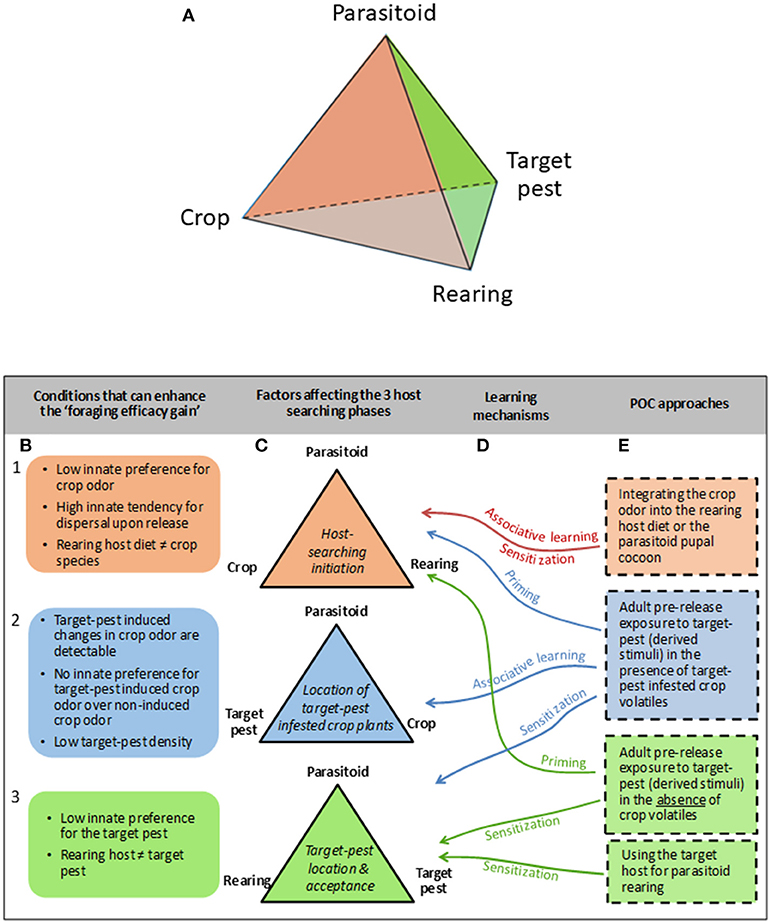
Figure 1. Tetrahedron depicturing the interplay between the four main factors affecting parasitoid efficacy for target-pest control (A). Each side of this tetrahedron corresponds to a different host-searching phase depicted by different colors (C). In particular, the “host-searching initiation” phase is marked by the orange color; the “location of target pest infested crop plants” phase is marked by the blue color that is at the back of the tetrahedron and is not visible on the (A); and the “target-pest location and acceptance” phase is marked in green. Each host-searching phase can be associated with specific conditions that can enhance the “foraging efficacy gain” (B), and can potentially be affected by different parasitoid olfactory conditioning (POC) approaches (E) mediated by associative and/or non-associative learning mechanisms (D).
The initiation of host searching behavior depends on the interplay between the parasitoid, the crop and the parasitoid rearing method (Figure 1C1). When the parasitoid has a high innate tendency for dispersal upon release, and/or a low innate preference for the crop odor, POC is expected to enhance the initiation of host searching behavior most (Figure 1B1). Integrating the crop odor into the rearing host diet or impregnating the parasitoid pupal case with the crop odor (Figure 1E1) may improve parasitoid retention in the crop and their motivation to search for hosts upon release through pre-adult or early-adult associative learning or sensitization. Moreover, pre-release exposure of adult parasitoids to target-pest (derived stimuli), either in the presence (Figure 1E2) or absence (Figure 1E3) of (target-pest infested) crop volatiles may also enhance the initiation of host searching behavior through priming.
Location of target-pest infested crop plants within the crop environment depends on the interplay between the parasitoid, the target-pest and the crop (Figure 1C2), and can be enhanced by adult pre-release exposure to target-pest (derived stimuli) in the presence of target-pest infested crop volatiles (Figure 1E2). Only when the target-pest induces a change in the volatile blend emitted by the crop plant that is detectable by the parasitoid, the parasitoid can use HIPVs to locate a target-pest infested plant. Moreover, the “foraging efficacy gain” increases when naive parasitoids do not have an innate preference for the target-pest induced crop odor over the non-induced crop odor. Finally, the chance of locating a host-infested plant through directed search resulting from associative learning will increase compared to random search when pest densities become lower (Figure 1B2).
Lastly, the location of the target-pest itself after the arrival of the parasitoid on the target-pest infested plant, as well as the acceptance of the target-pest for egg-laying, will depend on the interplay between the parasitoid species, the parasitoid rearing method and the target-pest species (Figure 1B3). When the target-pest represents a non-preferred host (Figure 1B3), pre-release exposure of adult parasitoids to the target-pest (or target-pest derived stimuli), either or not in the presence of (target-pest infested) crop volatiles, may increase target-pest location and acceptance through sensitization (Figures 1E2,3). Changing the host used in the rearing method for the non-preferred target-host may also enhance target-pest location and acceptance through early-adult sensitization (Figure 1E3).
Thus, the host location phase that contributes most to the foraging target-pest gain should co-determine the POC approach. Moreover, the POC approach as well as its technical implementation will determine the strength, duration and stability of the learning-induced behavioral change, which is directly related to the memory type that is formed. Memory formation following a learning event can be divided into different types or stages (Hoedjes et al., 2011). So-called short-term memory (STM) and mid-term memory (MTM) serve to store information temporally. Depending on the subsequent occurrence of conflicting or confirming information these memory forms usually wane within minutes to hours (STM), or hours to days (MTM). Long-term memory (LTM) is the most durable and resistant to extinction. Especially when parasitoids are released into a crop environment with low target-pest density, memory durability, and resistance to extinction in the face of unrewarding experiences may be very important for successful location of a target-pest infested plant. Finally, the four main factors (parasitoid, crop, target-pest species, and parasitoid rearing method) may all be influenced by multiple other factors but this goes beyond the scope of this perspective paper.
The Technical Implementation of POC – Costs and Benefits
In addition to the potential impact of POC on parasitoid foraging efficacy, the costs and benefits of POC should be weighed when deciding on the POC approach and its technical implementation. There may be direct costs (labor, ingredients and spatial requirements) and indirect costs (potential risks) involved in the POC method. These potential risks are context-dependent. For example, when POC takes place at the release site, using living pest insects as a reward in the conditioning procedure can constitute a contamination risk. When POC takes place at the manufacturer's site, economic risks may arise from an increased product diversification in relation to difficult-to-predict market demands. For parasitoid species that are expensive to rear, the benefits of POC will more quickly outweigh its costs. Furthermore, POC approaches can be applicable at all scales of augmentative biological control (greenhouses, small, and large fields). Below we discuss the different approaches to POC in relation to their technical implementation, potential impact, and direct and indirect costs along the lines of “what,” “how,” “where” and “when.”
With “what” we mean the characteristics of the parasitoids that will undergo POC. The learning rate of the parasitoid, i.e., the number of experiences that is needed to form LTM, is an important determinant of a successful implementation of POC. Depending on the natural circumstances in which parasitoid learning has evolved, a higher or a lower learning rate may be most adaptive (Stephens, 1993; Dukas and Duan, 2000; Dukas, 2008; Hoedjes et al., 2011). This has resulted in, often strong, between-species (e.g. Smid et al., 2007) as well as within-species (van den Berg et al., 2011; Koppik et al., 2015) variation in learning rate. Selection for a higher learning rate within a single parasitoid species is possible (van den Berg et al., 2011; Liefting et al., 2018) and its feasibility for improving the efficacy of POC should be further explored. Apart from genetic characteristics, the parasitoid developmental stage, age and physiological state can also affect learning rate. Parasitoid developmental stage will dictate the possible approaches to POC (Figure 1E). The relationship between parasitoid adult age and learning ability may depend on the learning mechanism involved. For example, Cotesia congregata females are only receptive to sensitization for plant odors for a few hours after emergence (Kester and Barbosa, 1991), whereas Honda and Kainoh (1998) found the associative learning ability of female Ascogaster reticulatus to be much lower during the first day after emergence when these parasitoids are not yet able to oviposit. Feeding status can also impact parasitoid responses to conditioned odors indicative of host-presence (Takasu and Lewis, 1993), but less is known about the influence of parasitoid satiation level during POC on subsequent memory formation for odors indicative of host presence and/or—identity (Siekmann et al., 2004; Tertuliano et al., 2004).
With “how” we mean the technical implementation of POC, covering aspects such as the conditioning approach, the duration and number of conditioning trials, the number of parasitoids that are conditioned in unison, as well as the characteristics of the rewarding stimulus and the conditioned stimulus. The amount of labor involved is tightly linked to the number of parasitoids that can be conditioned simultaneously, as well as to the complexity of the conditioning procedure, which in turn determines the number and time duration of the manual operations as well as the possibilities for automation.
Of all the POCapproaches outlined in Figure 1E, parasitoid pre-release exposure to the target-pest (derived stimuli) in the presence of target-pest infested crop volatiles is expected to have the highest impact on parasitoid foraging efficacy and target-pest control, because it may enhance all phases of the host searching process, and because it may induce LTM. At the same time, it may also be the most difficult to implement POC method. Especially when implemented at the grower's site, it will often be preferable to avoid the use of living target-pests. POC will then require both an adequate alternative reward as well as a stimulus that resembles the target-pest induced crop volatiles. Pest-derived stimuli such as artificial fecal pellets containing the reinforcing host recognition kairomone (Lewis and Martin, 1990), honeydew or scales may be used as an alternative reward, but are expected to act as a weaker reinforcer compared to an actual oviposition experience into a host-insect (Takasu and Lewis, 2003; Schurmann et al., 2012; Koppik et al., 2015). This potential drawback emphasizes the importance of selecting for parasitoids with a high learning rate. Artificially providing the correct HIPV blend during POC may pose a potentially more difficult challenge. One option to mimic HIPVs may be the addition of those volatile compounds that are enhanced by target-pest feeding to an intact crop plant. Another option may be the addition of elicitors of plant defensive pathways (Guo et al., 2013; Mack et al., 2013; e.g., Dinh et al., 2013; such as the plant hormones jasmonic acid or salicylic acid and/or enzymes from host saliva/regurgitant), either or not in combination with mechanically damaging a crop plant. It may not be necessary to mimic the complete volatile blend, as parasitoids are expected to generalize among non-functional differences in volatile blend composition resulting both from plant developmental and from environmental factors (Vet et al., 1998). When the composition of the volatile blend used in POC will more closely resemble the volatile composition of a target-pest infested plant than the volatile composition of a non-infested plant, parasitoids could be given a “head-start,” enabling them to further refine their odor preferences during foraging (Geervliet et al., 1998; Vet et al., 1998).
With “where” we mean the site where POC is carried out (i.e. at the manufacturer's site or at the release site), and with “when” we mean the time in between POC and release into the crop environment. Whenever POC takes place at the manufacturer's site, the effect of the conditions the parasitoids experience during shipment (such as the duration of transportation, abiotic conditions, chance of physical damage etc.) on memory retention should be assessed. Thermal or physical stress experienced during shipment may have a negative effect on memory retention (Margulies et al., 2005; van den Berg et al., 2011; Abram et al., 2015). Moreover, if shipment takes a long time it would be desirable that parasitoids form LTM that will not vanish during transportation (Hoedjes et al., 2011). When parasitoids are shipped as pupae, POC of adults can only take place at the release site. This has the benefit of the target crop already being present, which is more difficult and costly to realize at the manufacturer's site, especially when the parasitoids can be used in many different cropping systems. When the target-pest is already present in the crop, adapting the release strategy by confining the parasitoids for some time on target-pest infested plants before allowing them to disperse further may be sufficient to enhance their efficacy. However, when released as a preventative strategy, it will be preferable to resort to alternative rewards and artificial ways of mimicking the target-pest induced crop odor. In those cases, the manufacturer can provide the end-user not only with the parasitoid pupae, but also with a “POC package” that contains an alternative reward as well as HIPV containing pellets or HIPV elicitors.
Research Approaches for the Development of POC Methods
When facing the challenge of developing an optimal POC method for a specific combination of parasitoid species, target-pest and crop environment, it may be worthwhile to structure different types of experiments along a few clear research lines. Here we propose a structure of three main research lines, with a first research line focusing on the behavior of naive parasitoids, a second research line assessing—and possibly improving—parasitoid learning capability, and a third research line focusing on the technical implementation of POC.
The aim of the first research line would be to identify the potential for improving parasitoid efficacy during each of the three host searching phases by studying the behavior of naive parasitoids. Experiments can be carried out to determine: (a) the degree to which the parasitoid innately responds toward the crop odor as well as the parasitoids' innate tendency for dispersal upon release, (b) whether the parasitoid innately prefers the odor of the target-pest induced crop over non-induced crop odor and whether the parasitoid can distinguish between these odors at different target-pest densities, and (c) the extent to which the parasitoid is innately attracted toward target-pest derived cues and accepts target-pests for parasitization.
The main aim of the second research line would be to assess the impact of different types of learning (associative learning, priming, and sensitization) in combination with the parasitoids' developmental stage on the strength and duration of the learned response, and – in the case of associative learning – to determine the number of conditioning trials required for the establishment of long-term memory. The results from the first research line can be used to determine the focus of this second research line. If multiple conditioning trials result in a significant enhancement of the strength and duration of the learned response, it may be worthwhile to further investigate the possibilities for improvement of the learning rate through an artificial selection program.
The main aim of the third research line would be to identify a POC method that leads to the highest possible parasitoid efficacy gain at the lowest direct and indirect costs. Depending on the POC approach chosen based on the results of the other research lines, it may be important to assess the effects of alternative rewards and/or artificial ways of mimicking target-pest induced crop volatiles in POC on the strength and duration of the parasitoids' learned response. Moreover, determining the maximum number of parasitoids that can be conditioned in unison, as well as the best timing for conditioning in relation to parasitoid release are important pieces of information for the optimization of a POC method. In case the parasitoids are conditioned at the manufacturer's site, it will also be important to assess the impact of shipment conditions on memory retention.
Conclusions
The ability of parasitoids to optimize their foraging behavior through learning has been widely demonstrated in controlled laboratory conditions. This offers a great potential for optimizing the use of parasitoids in augmentative biological pest control. Especially when a parasitoid species is expensive to rear, POC may quickly pay off. We will therefore need more studies that systematically assess the potential impact of POC on parasitoid efficacy for biological pest control, as well as the possibilities for optimizing parasitoid learning rate and POC implementation for a series of commercially important parasitoid-pest-crop combinations.
Author Contributions
HK and LV conceived the original idea. HK and OK developed the main conceptual ideas and wrote the first draft of the manuscript. All authors provided critical feedback and contributed to the final manuscript.
Conflict of Interest Statement
The authors declare that the research was conducted in the absence of any commercial or financial relationships that could be construed as a potential conflict of interest.
References
Abram, P. K., Cusumano, A., Peri, E., Brodeur, J., Boivin, G., and Colazza, S. (2015). Thermal stress affects patch time allocation by preventing forgetting in a parasitoid wasp. Behav. Ecol. 26, 1326–1334. doi: 10.1093/beheco/arv08
Dinh, S. T., Baldwin, I. T., and Galis, I. (2013). The herbivore elicitor-regulated1 gene enhances abscisic acid levels and defenses against herbivores in Nicotiana attenuata plants. Plant Physiol. 162, 2106–2124. doi: 10.1104/pp.113.221150
Dukas, R. (2008). Evolutionary biology of insect learning. Ann. Rev. Entomol. 53, 145–160. doi: 10.1146/annurev.ento.53.103106.093343
Dukas, R., and Duan, J. J. (2000). Potential fitness consequences of associative learning in a parasitoid wasp. Behav. Ecol. 11, 536–543. doi: 10.1093/beheco/11.5.536
Geervliet, J. B. F., Vreugdenhil, A. I., Dicke, M., and Vet, L. E. M. (1998). Learning to discriminate between infochemicals from different plant-host complexes by the parasitoids Cotesia glomerata and C-rubecula. Entomol. Exp. Et Appl. 86, 241–252. doi: 10.1046/j.1570-7458.1998.00286.x
Giunti, G., Canale, A., Messing, R. H., Donati, E., Stefanini, C., Michaud, J. P., et al. (2015). Parasitoid learning: current knowledge and implications for biological control. Biol. Control 90, 208–219. doi: 10.1016/j.biocontrol.2015.06.007
Gross, H. R., Lewis, W. J., Jones, R. L., and Nordlund, D. A. (1975). Kairomones and their use for management of entomophagous insects: III. Stimulation of Trichogramrna achaeae, T. pretiosum, and Microplitis croceipes with host-seeking stimuli at time of release to improve their efficiency. J. Chem. Ecol. 1, 431–438.
Guo, H. J., Wielsch, N., Hafke, J. B., Svatos, A., Mithofer, A., and Boland, W. (2013). A porin-like protein from oral secretions of Spodoptera littoralis larvae induces defense-related early events in plant leaves. Insect Biochem. Mol. 43, 849–858. doi: 10.1016/j.ibmb.2013.06.005
Hare, J. D., and Morgan, D. J. W. (1997). Mass-priming aphytis: behavioral improvement of insectary-reared biological control agents. Biological. Control. 10, 207–214. doi: 10.1006/bcon.1997.0565
Hare, J. D., Morgan, D. J. W., and Nguyun, T. (1997). Increased parasitization of California red scale in the field after exposing its parasitoid, Aphytis melinus, to a synthetic kairomone. Entomol. Exp. Et Appl. 82, 73–81. doi: 10.1046/j.1570-7458.1997.00115.x
Hoedjes, K. M., Kruidhof, H. M., Huigens, M. E., Dicke, M., Vet, L. E. M., and Smid, H. M. (2011). Natural variation in learning rate and memory dynamics in parasitoid wasps: opportunities for converging ecology and neuroscience. Proc. R Soc. B-Biol. Sci. 278, 889–897. doi: 10.1098/rspb.2010.2199
Honda, T., and Kainoh, Y. (1998). Age-related fecundity and learning ability of the egg-larval parasitoid Ascogaster reticulatus Watanabe (Hymenoptera : Braconidae). Biol. Control. 13, 177–181. doi: 10.1006/bcon.1998.0657
Ishii, Y., and Shimada, M. (2010). The effect of learning and search images on predator-prey interactions. Popul. Ecol. 52, 27–35. doi: 10.1007/s10144-009-0185-x
Kester, K. M., and Barbosa, P. (1991). Postemergence learning in the insect parasitoid, Cotesia congregata (Say) (Hymenoptera, Braconidae). J. Insect Behav. 4, 727–742. doi: 10.1007/Bf01052227
Koppik, M., Hoffmeister, T. S., Brunkhorst, S., Kieß, M., and Thiel, A. (2015). Intraspecific variability in associative learning in the parasitic wasp Nasonia vitripennis. Anim. Cogn. 18, 593–604. doi: 10.1007/s10071-014-0828-y
Lewis, W. J., and Martin, W. R. (1990). Semiochemicals for use with parasitoids - status and future. J. Chem. Ecol. 16, 3067–3089. doi: 10.1007/bf00979613
Liefting, M., Hoedjes, K. M., Le Lann, C., Smid, H. M., and Ellers, J. (2018). Selection for associative learning of color stimuli reveals correlated evolution of this learning ability across multiple stimuli and rewards Evolution 72, 1449–1459. doi: 10.1111/evo.13498
Mack, L., Gros, P., Burkhardt, J., and Seifert, K. (2013). Elicitors of tansy volatiles from cotton leafworm larval oral secretion. Phytochemistry 96, 158–169. doi: 10.1016/j.phytochem.2013.08.007
Margulies, C., Tully, T., and Dubnau, J., (2005). Deconstructing memory in Drosophila. Curr. Biol. 15, R700–R713. doi: 10.1016/j.cub.2005.08.024
Clavijo McCormick, A., Unsicker, S. B., and Gershenzon, J. (2012). The specificity of herbivore-induced plant volatiles in attracting herbivore enemies. Trends Plant Sci. 17, 303–310. doi: 10.1016/j.tplants.2012.03.012
Nieberding, C. M., Van Dyck, H., and Chittka, L. (2018). Adaptive learning in non-social insects: from theory to field work, and back. Curr. Opin. Insect Sci. 27, 75–81. doi: 10.1016/j.cois.2018.03.008
Papaj, D. R., and Prokopy, R. J. (1989). Ecological and evolutionary aspects of learning in phytophagous insects. Ann. Rev. Entomol. 34, 315–350.
Papaj, D. R., and Vet, L. E. (1990). Odor learning and foraging success in the parasitoid, Leptopilina heterotoma. J. Chem. Ecol. 16, 3137–3150. doi: 10.1007/bf00979616
Pilkington, L. J., Messelink, G., van Lenteren, J. C., and Le Mottee, K. (2010). “Protected Biological Control” - biological pest management in the greenhouse industry. Biol. Control 52, 216–220. doi: 10.1016/j.biocontrol.2009.05.022
Schurmann, D., Sommer, C., Schinko, A. P. B., Greschista, M., Smid, H., and Steidle, J. L. M. (2012). Demonstration of long-term memory in the parasitic wasp Nasonia vitripennis. Entomol. Exp. Et Appl. 143, 199–206. doi: 10.1111/j.1570-7458.2012.01253.x
Siekmann, G., Keller, M. A., and Tenhumberg, B. (2004). The sweet tooth of adult parasitoid Cotesia rubecula: Ignoring hosts for nectar? J. Insect Behav. 17, 459–476. doi: 10.1023/B:JOIR.0000042535.76279.c7
Smid, H. M., and Vet, L. E. (2016). The complexity of learning, memory and neural processes in an evolutionary ecological context. Curr. Opin. Insect Sci. 15, 61–69. doi: 10.1016/j.cois.2016.03.008
Smid, H. M., Wang, G., Bukovinszky, T., Steidle, J. L., Bleeker, M. A. K., van Loon, J. J. A., et al. (2007). Species-specific acquisition and consolidation of long-term memory in parasitic wasps. Proc. R Soc. B-Biol. Sci. 274, 1539–1546. doi: 10.1098/rspb.2007.0305
Stephens, D. W. (1993). “Learning and behavioral ecology: incomplete information and environmental predictability,” in Insect Learning: Ecological and Evolutionary Perspectives, eds. D.R. Papaj and A.C. Lewis. (New York, NY: Chapman and Hall), 195–218.
Takasu, K., and Lewis, W. J. (1993). Host-foraging and food-foraging of the parasitoid Microplitis croceipes- learning and physiological state effects. Biol. Control. 3, 70–74. doi: 10.1006/bcon.1993.1011
Takasu, K., and Lewis, W. J. (2003). Learning of host searching cues by the larval parasitoid Microplitis croceipes. Entomol. Exp. Et Appl. 108, 77–86. doi: 10.1046/j.1570-7458.2003.00070.x
Tertuliano, M., Olson, D. M., Rains, G. C., and Lewis, W. J. (2004). Influence of handling and conditioning protocol on learning and memory of Microplitis croceipes. Entomol. Exp. et Appl. 110, 165–172. doi: 10.1111/j.0013-8703.2004.00132.x
Turlings, T. C. J., Wackers, F. L., Vet, L. E. M., Lewis, W. J., and Tumlinson, J. H. (1993). “Learning of host-finding cues by hymenopterous parasitoids,” in Insect Learning: Ecological And Evolutionary Perspectives, eds. D.R. Papaj and A.C. Lewis. (New York: Chapman and Hall), 51–78.
van den Berg, M., Duivenvoorde, L., Wang, G., Tribuhl, S., Bukovinszky, T., Vet, L. E. M., et al. (2011). Natural variation in learning and memory dynamics studied by artificial selection on learning rate in parasitic wasps. Anim. Behav. 81, 325–333. doi: 10.1016/j.anbehav.2010.11.002
van Lenteren, J. C., Bolckmans, K., Kohl, J., Ravensberg, W. J., and Urbaneja, A. (2018). Biological control using invertebrates and microorganisms: plenty of new opportunities. Biocontrol 63, 39–59. doi: 10.1007/s10526-017-9801-4
Vet, L. E. M., De Jong, A. G., Franchi, E., and Papaj, D. R. (1998). The effect of complete versus incomplete information on odour discrimination in a parasitic wasp. Anim. Behav. 55, 1271–1279. doi: 10.1006/anbe.1997.0686
Vet, L. E. M., and Dicke, M. (1992). Ecology of infochemical use by natural enemies in a tritrophic context. Ann. Rev. Entomol. 37, 141–172. doi: 10.1146/annurev.en.37.010192.001041
Vet, L. E. M., and Groenewold, A. W. (1990). Semiochemicals and learning in parasitoids. J. Chem. Ecol. 16, 3119–3135. doi: 10.1007/Bf00979615
Vet, L. E. M., and Papaj, D. R. (1992). Effects of experience on parasitoid movement in odor plumes. Physiol. Entomol. 17, 90–96. doi: 10.1111/j.1365-3032.1992.tb00994.x
Vet, L. E. M., Wackers, F. L., and Dicke, M. (1991). How to hunt for hiding hosts - the reliability-detectability problem in foraging parasitoids. Netherlands J. Zool. 41, 202–213.
Vinson, S. B. (1976). Host selection by insect parasitoids. Ann. Rev. Entomol. 21, 109–133. doi: 10.1146/annurev.en.21.010176.000545
Wajnberg, E., and Colazza, S. (2013). Chemical Ecology of Insect Parasitoids. London: Wiley-Blackwel.
White, J. A., and Andow, D. A. (2007). Foraging for intermittently refuged prey: theory and field observations of a parasitoid. J. Anim. Ecol. 76, 1244–1254. doi: 10.1111/j.1365-2656.2007.01299.x
Keywords: associative learning, foraging behavior, natural enemies, parasitoids, searching efficacy
Citation: Kruidhof HM, Kostenko O, Smid HM and Vet LEM (2019) Integrating Parasitoid Olfactory Conditioning in Augmentative Biological Control: Potential Impact, Possibilities, and Challenges. Front. Ecol. Evol. 7:84. doi: 10.3389/fevo.2019.00084
Received: 19 November 2018; Accepted: 05 March 2019;
Published: 28 March 2019.
Edited by:
Paul-André Calatayud, Institut de Recherche Pour le Développement (IRD), FranceReviewed by:
Sylvia Anton, Institut National de la Recherche Agronomique (INRA), FranceAnne Marie Cortesero, University of Rennes 1, France
Copyright © 2019 Kruidhof, Kostenko, Smid and Vet. This is an open-access article distributed under the terms of the Creative Commons Attribution License (CC BY). The use, distribution or reproduction in other forums is permitted, provided the original author(s) and the copyright owner(s) are credited and that the original publication in this journal is cited, in accordance with accepted academic practice. No use, distribution or reproduction is permitted which does not comply with these terms.
*Correspondence: H. Marjolein Kruidhof, marjolein.kruidhof@wur.nl
Olga Kostenko, o.kostenko@nioo.knaw.nl
†These authors have contributed equally to this work