- 1Research Institute for Sport and Exercise, University of Canberra, Canberra, ACT, Australia
- 2Australian Centre for Health Services Innovation and Centre for Healthcare Transformation, School of Public Health and Social Work, Faculty of Health, Queensland University of Technology, Brisbane, QLD, Australia
- 3Gene Regulation and Translational Medicine Laboratory, Immunology Department, QIMR Berghofer Medical Research Institute, Brisbane, QLD, Australia
The impaired effector function of exhausted and senescent T cells is implicated in cancer progression and inadequate vaccine responses. Exercise has been shown to improve cancer therapy and vaccine efficacy, most likely by improving immune function. However, given inconsistent terminology and definitions, the interactions between exercise and exhausted and senescent T cells remain unclear. We therefore performed a systematic review to investigate the effect of exercise on senescent and exhausted CD8+ T cell populations clearly defined by protein surface markers. Thirty articles were included, with the majority (n = 24) reporting senescent T cell populations defined according to a variety of surface markers. Repeated exercise was shown to be beneficial through limiting the accumulation of senescent and exhausted CD8+ T cells. This outcome is likely related to exercise-induced preferential mobilization of senescent T cells promoting apoptosis in the peripheral blood compartment. Future studies need to determine the clinical relevance of this effect in cancer prevention and vaccine efficacy. Data regarding exercise and exhausted T cells are limited due to a lack of available high-quality studies. Future studies require the control of confounding variables such as sex and cytomegalovirus (CMV) status, and consistent definitions of exhausted and senescent T cell populations to improve comparisons between studies and interventions.
Introduction
As drivers of the adaptive immune response, CD8+ T cells are important actors in cancer prevention and vaccine efficiency. However, exhausted or senescent CD8+ T cells have impaired effector function, thereby contributing to cancer development and decreasing vaccine efficiency (McElhaney et al., 2016; Huff et al., 2019). Exhausted and senescent T cells are present in the tumor microenvironment and in older adults, who typically have impaired vaccine responses (Simpson et al., 2012; Crespo et al., 2013). The terminology and definitions for senescent and exhausted CD8+ T cells are yet to be finalized resulting in a lack of clarity in research for these cell types. In the past, senescent cells have been referred to as late-stage, terminally, or highly differentiated T cells (Turner, 2016; Duggal et al., 2019). Adding to the confusion, the terms “exhausted” and “senescent” T cells have been used interchangeably (Bigley et al., 2013; Pawelec, 2019). Exhausted and senescent T cells have different origins, identifications (protein surface markers), and functional ability. Exhausted T cells arise from excessive and prolonged stimulation of the T cell receptor (TCR) and the action of inflammatory cytokines, which progressively suppress T cell effector functions (Pawelec, 2019). In contrast, senescent T cells arise through aging and/or chronic infection (Turner, 2016). The two cell types can be distinguished by protein surface marker expression and other features, as outlined in Table 1 (Crespo et al., 2013; Huff et al., 2019). Although different cell types, exhausted and senescent CD8+ T cells share similarities. For example, cytomegalovirus (CMV) infection can drive the development of both cell types, KLRG1+ is a common surface marker, and both subtypes have a reduced ability to produce cytokines (Simpson et al., 2016; Huff et al., 2019). Some researchers propose there are benefits to senescent T cells such that they maintain their functionality as anti-CMV immunosurveillance by being prevented from further expansion, and lost by clonal attrition (Pawelec, 2019). Similarly, exhausted T cells could be advantageous in autoimmune disease and organ transplantation (Pawelec, 2019). However, regarding cancer and vaccine efficiency evidence indicates that senescent and exhausted T cells are a burden (McElhaney et al., 2016; Huff et al., 2019).
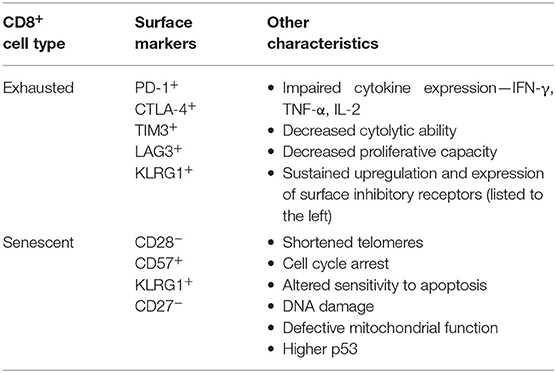
Table 1. Typical surface markers and established characteristics of exhausted and senescent CD8+ T cells.
Given the pathophysiological consequences of T cell senescence and exhaustion, efforts are underway to overcome impaired exhausted and senescent T cell function. One approach has been to exploit epigenetic mechanisms to improve immunotherapy and vaccine efficiency (Dan et al., 2020). Epigenetics is the study of potentially heritable changes in gene expression without changes in the DNA base sequence (Berger et al., 2009), and these dynamic mechanisms can be influenced by many environmental factors (Ling and Ronn, 2014). One important environmental epigenetic modifier is exercise, and recognition of its importance in influencing outcomes is reflected in its prescription for treatment of at least 26 different diseases or conditions, including psychiatric, neurological, metabolic, cardiovascular, pulmonary, musculoskeletal, and cancer (Pedersen and Saltin, 2015). There has been little research on the effect of exercise on exhausted T cells, with greater focus on its effect on senescent T cells. In their 2012 review, Simpson et al. (2012) reported that many cross-sectional studies demonstrate that exercise reduces the numbers of senescent T cells and increases T cell proliferation. However, there is conflicting evidence on the extent to which exercise influences T cell proliferation, most likely related to methodological variations in both the exercise interventions and definitions of senescent T cell populations in different studies (Simpson et al., 2012). The definition of senescent (and exhausted) T cells has been refined in recent years, and using the current definitions presented in Table 1, uniform studies could be designed to define the impact of exercise on these populations (Huff et al., 2019). Studying epigenetics in the clinic, laboratory, and field should be useful for understanding how exercise influences these cells, the effectiveness of various interventions, and ultimately clinical outcomes.
The aim of this systematic review was to aggregate current knowledge on the effect of exercise on exhausted and senescent CD8+ T cells, which are implicated in cancer progression and vaccine responses. We sought to differentiate the effects of acute (single bouts) exercise with the cumulative effects of repeated exercise on these types of T cells. In doing so, we identify areas requiring further investigation, optimal experimental approaches for these investigations, and how rigorous definition of cell populations and outcomes may promote exercise as an adjunct therapy for cancer and vaccines by limiting exhausted and senescent T cell numbers.
Methods of Systematic Review
Protocol
The systematic review followed the Preferred Reporting Items for Systematic Reviews and Meta-Analyses (PRISMA) guidelines for systemic reviews (Moher et al., 2009).
Search Strategy
The electronic databases of PubMed, Embase, and Web of science were searched between January 2010 and 11 April 2021 (last date searched). Subject headings and keywords such as “T cell,” “senescence,” “exhaustion,” specific biomarkers, and “exercise” were combined in the search. The detailed search strategy is outlined in Supplementary Table 1.
Eligibility Criteria
The PICOS framework was used to define the eligibility criteria. Studies that met the following criteria were included: (1) P (population): adults (over 16 years old), in vivo human studies, no restriction on sex or physical activity level; (2) I (intervention/exposure): acute and repeated exercise, physical activity level, or sport; (3) C (comparison): control group or baseline data; (4) O (outcomes): reported on the number/proportion of a protein-surface-marker-defined senescent or exhausted CD8+ T cell population/s; (5) S (study designs): cross-sectional, longitudinal, intervention, randomized controlled trial (RCT), or randomized cross-over trial (RCOT). Studies with the following characteristics were excluded: animal studies, reviews, abstracts only, case studies, commentary pieces, non-English studies, or studies investigating participants under the age of 16 years old, have immune system comorbidities, have contraindications to exercise therapy, or are pregnant. If more than one article reported on the same cohort population, only one study was included based on relevance and recency.
Study Selection
Article titles and abstracts were first screened, followed by screening of the full text to confirm that the study fit the eligibility criteria. Two authors (TD and DP) independently conducted the entire screening process, and discrepancies were resolved through discussion.
Data Collection
The first author, year published, study design, defined exhausted or senescent T cell population/s, cohort description, exercise intervention (if applicable), data collection, and results on the impact of exercise on the exhausted or senescent T cell population/s were collated and presented.
Quality Assessment
National Institutes of Health (NIH) quality assessment (QA) tools were employed to evaluate the included studies (National Heart Lung and Blood Institute, 2021). Specifically, the NIH QA tool for observational cohort and cross-sectional studies, and the NIH QA tool for a before-after (pre-post) study with no control group, were adapted to create a single QA tool (see Supplementary Table 2) as several included studies featured both cross-sectional and intervention arms. In addition, RCT and RCOT studies were assessed using the NIH QA tool for controlled intervention studies (see Supplementary Table 3). All QA tool outcomes were identical to permit comparison of different study types, and assessment of quality was determined as either poor, fair, or good.
Results
Overview of Included Studies
The search yielded 369 articles, without duplicates. Titles and abstracts were screened for suitability which eliminated 307 ineligible articles, the majority of which were reviews and conference abstracts. The full text of 62 studies were screened to assess their eligibility, and 32 studies were eliminated due to the following reasons: not investigating a senescent or exhausted CD8+ T cell population defined by protein surface markers (n = 23); no exercise intervention or exposure level was studied (n = 5); participant cohort was the same as an already included study (n = 3); or no control group or baseline data was reported (n = 1). Figure 1 illustrates the study selection process. A final total of 30 included studies investigated the impact of exercise on senescent (n = 24) and/or exhausted (n = 7) CD8+ T cells—one study investigated both populations.
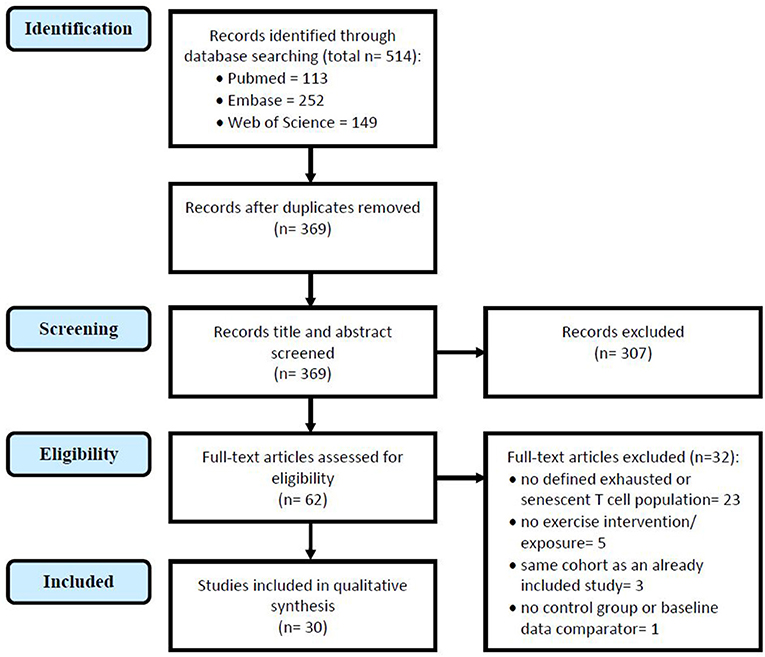
Figure 1. Study selection in accordance with the Preferred Reporting Items for Systematic Reviews and Meta-Analyses (PRISMA) guidelines for systemic reviews (Moher et al., 2009), the above flowchart illustrates the selection process of studies in this review.
The type of study, protein surface marker/s used to define the CD8+ T cell population, cohort characteristics, data collection methods, exercise intervention details, and outcomes of the included studies are outlined in Table 2 (senescent T cells) and Table 3 (exhausted T cells). Briefly, the majority of included studies had features of cross-sectional and/or intervention analyses. Various protein surface markers were used to identify senescent and exhausted CD8+T cell populations, it is beyond the scope of this review to determine the optimal/most valid biomarker combination. The most commonly reported cohort characteristics were age, physical activity level, and sex. All included studies collected venous blood samples to investigate their aims and most (n = 15) reported VO2max (maximal aerobic capacity) scores as a measure of aerobic fitness. Acute exercise was more commonly investigated (n = 21) than repeated exercise completed over multiple days and/or weeks (n = 10). The types of acute exercise examined included: cycling (n = 14), running (n = 6), futsal game (n = 1), strength (n = 1), and swimming (n = 1). Resistance training (n = 3), cycling (n = 2), walking (n = 2), running (n = 2), swimming (n = 1), and triathlon training (n = 1) were studied as types of repeated exercise.
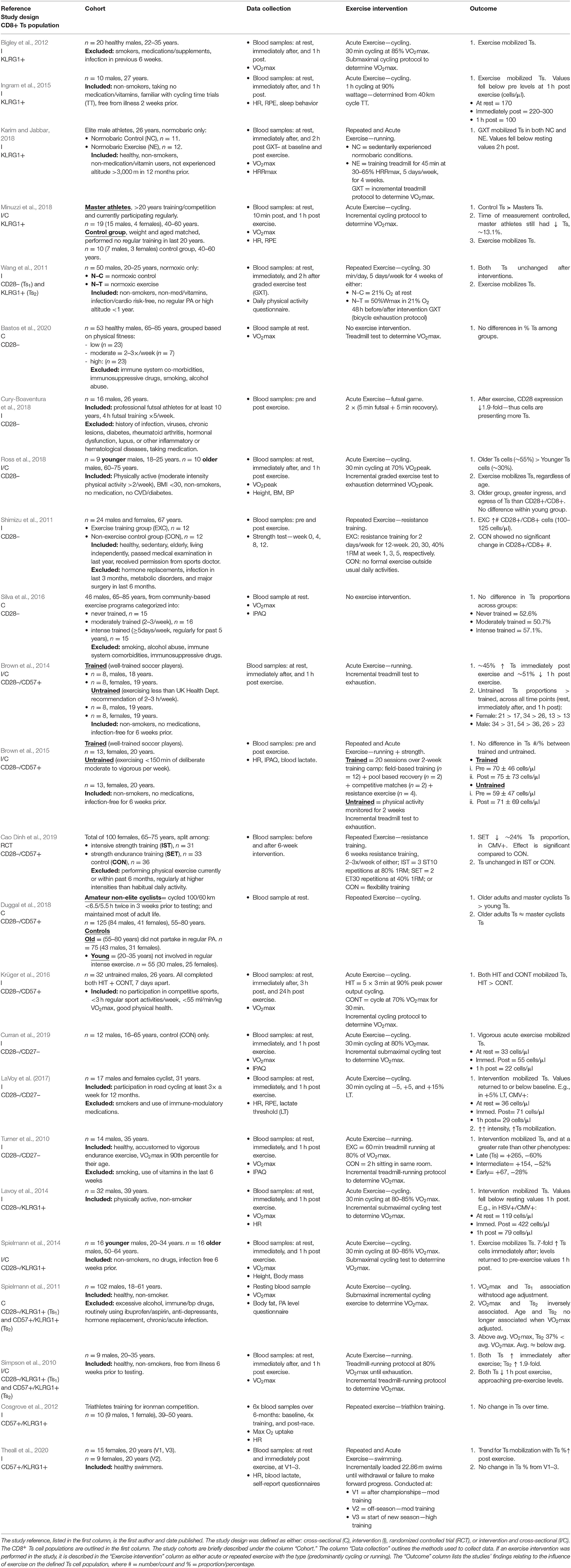
Table 2. Summary of results detailing the influence of exercise on senescent T (Ts) cells from studies included in this systematic review.
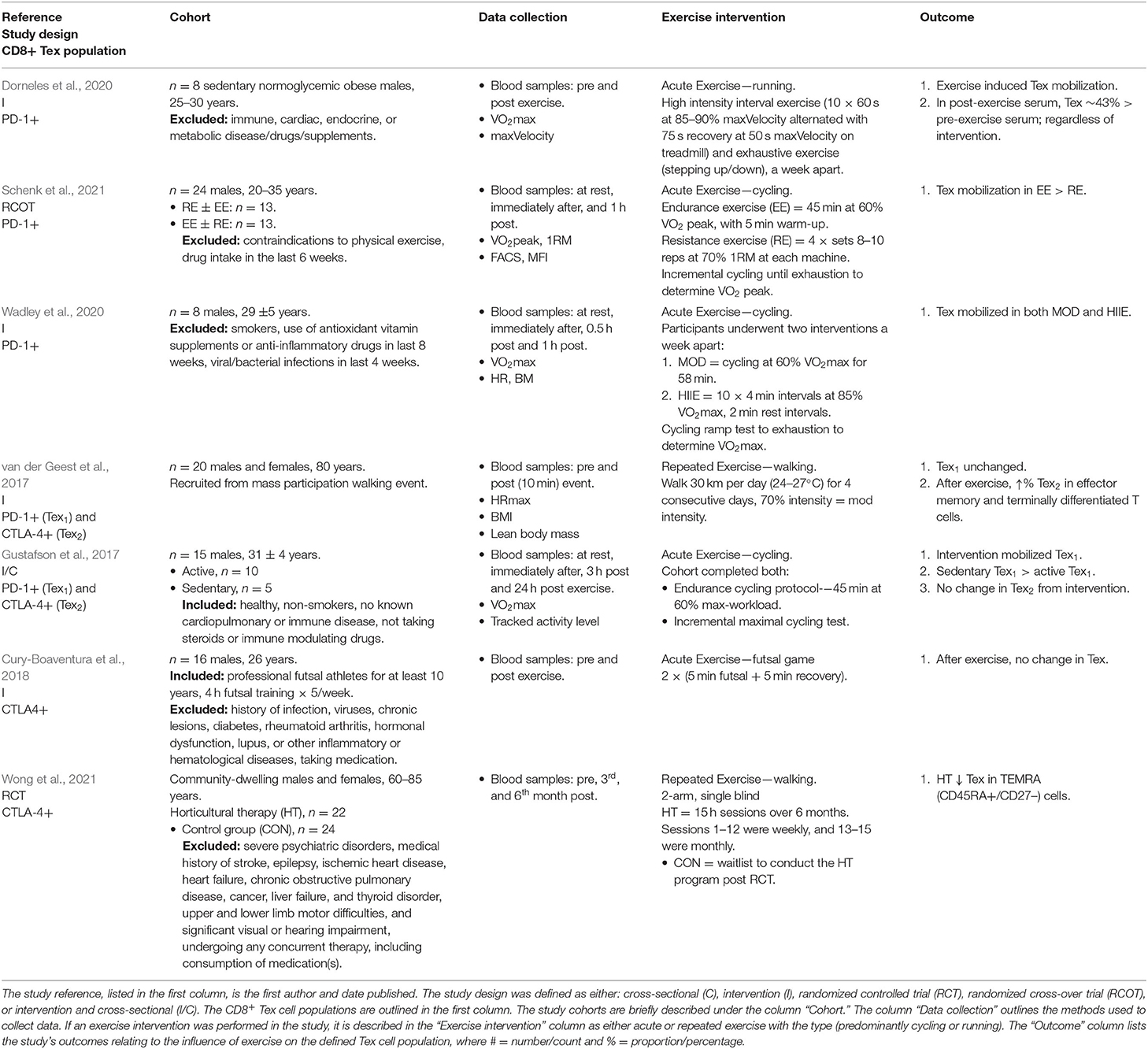
Table 3. Summary of results concerning the influence of exercise on exhausted T (Tex) cells from included studies in this systematic review.
Quality Assessment
National Institutes of Health (NIH) quality assessment (QA) tools were used to assess the quality of the included studies. The majority of studies were deemed high quality (n = 21), nine studies were rated moderate quality, and no studies were considered low quality. Common limitations included small sample size, absence of participant blinding, and lack of multiple outcome/exposure measurements. Among the moderate quality studies, additional limitations included only modest descriptions of interventions/exposures, exposure not measured prior to outcome, single level/amount of exposure examined, short timeframe for association between intervention/exposure and outcome, and inadequate statistical analysis, particularly in adjustment(s) for confounding variables. Supplementary Table 4 contains detailed scores for each included study.
Senescent CD8+ T Cells
Acute Exercise Induces Mobilization of Senescent CD8+ T Cells
Most studies investigating senescent CD8+ T cells after acute exercise reported that acute exercise mobilized senescent T cells into the periphery (n = 16). This common conclusion was demonstrated by a transient increase in senescent T cell numbers immediately after exercise, followed by numbers returning to pre-exercise levels approximately 1–3 h after exercise (Simpson et al., 2010; Turner et al., 2010; Wang et al., 2011; Bigley et al., 2012; Brown et al., 2014; Lavoy et al., 2014; LaVoy et al., 2017; Spielmann et al., 2014; Ingram et al., 2015; Krüger et al., 2016; Cury-Boaventura et al., 2018; Karim and Jabbar, 2018; Minuzzi et al., 2018; Ross et al., 2018; Curran et al., 2019; Theall et al., 2020). Two exceptions to this finding were in acute exercise studies that only measured senescent T cells at rest, and thus could not report mobilization (Spielmann et al., 2011; Bastos et al., 2020). Among the studies demonstrating mobilization, a variety of protein surface markers were used to define the senescent T cells including: KLRG1+ (n = 5); CD28− (n = 3); CD28−/CD57+ (n = 2); CD28−/CD27− (n = 3); CD28-/KLRG1+ (n = 3); and CD57+/KLRG1+ (n = 2). Aerobic exercise in the form of cycling (n = 10), running (n = 4), swimming (n = 1), and futsal (n = 1) were employed as the type of acute exercise. Cohort characteristics ranged from male to female, athletes to sedentary, and young to older adults. Overall, regardless of the protein surface markers used to define senescent T cell populations, and cohort characteristics such as sex, physical activity level, and age, acute aerobic exercise generally mobilized senescent CD8+ T cells.
One of the studies demonstrated that in an older cohort only, CD28− senescent T cells were preferentially mobilized in response to exercise compared to CD28+ T cells (Ross et al., 2018). Although a comparable study with a similar aged cohort, does not report this finding, their results (in the first figure under CMV seronegative individuals for direct comparison) illustrate a similar trend (Simpson et al., 2011). Both studies indicate that age does not impact the ingress and subsequent egress induced by acute exercise. However, CMV serostatus can influence exercise induced mobilization of senescent T cell such that CMV seropositive individuals have a greater ingress and egress of senescent T cells after acute exercise (Turner et al., 2010; Simpson et al., 2011; Bigley et al., 2012; Lavoy et al., 2014; LaVoy et al., 2017). Exercise intensity can also alter mobilization whereby greater exercise intensity resulted in greater mobilization of senescent T cell, and other T cell populations (Krüger et al., 2016; LaVoy et al., 2017). Therefore, under certain conditions such as advanced age, CMV seropositivity and high exercise intensity, senescent CD8+ T cells may be preferentially mobilized in response to acute exercise.
Increased Cardiorespiratory Fitness Can Protect Against Senescent CD8+ T Cells
Increased cardiorespiratory fitness can protect against the production/accumulation of senescent CD8+ T cells populations. Individuals with an above-average cardiorespiratory fitness measured by VO2max (47.3 ml/kg/min) had 37% fewer KLRG1+/CD57+ and KLRG1+/CD28− senescent T cells than individuals with an average or below-average VO2max (43.0 and 34.4 ml/kg/min, respectively) (Spielmann et al., 2011). A cross-sectional study demonstrated a similar result where master athletes had accumulated less KLRG1+ senescent T cells than aged-matched sedentary controls (Minuzzi et al., 2018). The VO2max of the master athletes (40.4 ml/kg/min), aged between 40 and 60 years old, was less than the above-average VO2max in the previous study, which included participants aged from 18 to 61 years; this variation likely relates to the known decline of VO2max with age (Rogers et al., 1990; Bradshaw et al., 2005; Spielmann et al., 2011; Minuzzi et al., 2018). However, the Spielmann at al. study demonstrated that the relationship between VO2max and senescent T cells withstood adjustment for age (Spielmann et al., 2011). In summary, several studies demonstrate a trend toward increased cardiorespiratory fitness protecting against the accumulation of senescent CD8+ T cells; however, more research is required to confirm this finding.
Endurance Resistance Training Can Reduce Senescent CD8+ T Cells in Sedentary Older Adults
Resistance training exercise interventions were shown to decrease senescent CD8+ T cells populations. Resistance training over a 6- and 12-week intervention reduced the proportion of CD28−/CD57+ and number of CD28− senescent T cells, respectively (Shimizu et al., 2011; Cao Dinh et al., 2019). The cohorts of both studies consisted of sedentary older adults aged 61–79 years. The 6-week intervention only altered CD28−/CD57+ senescent T cells in individuals performing strength endurance training, and not intensive strength training. The strength endurance training more closely resembled the 12-week intervention in the Shimizu et al. study (Shimizu et al., 2011; Cao Dinh et al., 2019). Therefore, repeated endurance resistance training appears to reduce senescent CD8+ T cells in sedentary older adults >60 years.
Cohort Characteristics Associated With Unchanged Senescent CD8+ T Cells
It is well-established that senescent T cells accumulate with age (Crespo et al., 2013), not surprisingly, studies with a young (under 30 years old) cohort typically do not report any differences in senescent T cell populations with exercise, as the senescent T cell populations are likely too small for meaningful changes to be detected (Wang et al., 2011; Karim and Jabbar, 2018; Theall et al., 2020). However, the two Brown et al. (2014, 2015) studies both contained a young cohort yet only one demonstrated a change in senescent T cells between trained and untrained individuals (Brown et al., 2014, 2015). The contrasting result is likely due to differences in physical activity level of the “untrained” individuals. The study which showed no change in senescent T cells included “untrained” individuals who were physically active for 3.6 hours per week, compared to 2.0 hours per week (results for females only for direct comparison) in the other study (Brown et al., 2014, 2015). Other studies consisting of physically fit cohorts demonstrated similar results where senescent T cells were unchanged after exercise (Cosgrove et al., 2012; Silva et al., 2016; Bastos et al., 2020). Overall, it appears that acute and/or repeated exercise resulting in no change to senescent CD8+ T cells is largely related to cohort characteristics such as young age and high physical fitness.
Long-Term Exercise May Maintain Senescent CD8+ T Cells Telomere Length
Senescent CD8+ T cells are known to have shorter telomeres, with cross-sectional data indicating that CD28+ cells have longer telomeres than CD28− T cells (Silva et al., 2016). Participants who had not engaged in training in the previous 5 years had ~15–20% shorter CD8+ and senescent T cell telomeres than those who had participated regularly in exercise and physical activity. Although the number of senescent CD8+ T cells was unchanged in this study, it appears that exercise may protect against senescence through the maintenance of telomere length (Silva et al., 2016).
The Influence of Repeated Exercise on Senescent CD8+ T Cells Impacts Cytokine Levels
The impact of repeated exercise on senescent CD8+ T cells appeared to extend beyond reducing cell numbers, and into restoring immune function mediated by cytokines (Wang et al., 2011). Interferon-γ (IFN-γ) levels increased, and interleukin-6 (IL-6) and plasma myeloperoxidase (MPO) levels decreased when senescent T cell numbers decreased. Furthermore, when senescent T cell numbers remained stable, these cytokine levels were also stable (Wang et al., 2011; Silva et al., 2016; Duggal et al., 2018). Therefore, the ability of repeated exercise to limit senescent CD8+ T cell accumulation appears to have functional consequences through alterations in blood cytokine concentrations.
Exhausted CD8+ T Cells
Acute Exercise Induces Mobilization of PD-1+ and May Not in CTLA-4+ CD8+ T Cells
Similar to senescent CD8+ T cell populations, acute exercise induced the mobilization of PD-1+ exhausted CD8+ T cells into the peripheral blood compartment (Gustafson et al., 2017; Dorneles et al., 2020; Wadley et al., 2020; Schenk et al., 2021). Furthermore, blood serum collected after acute exercise induced CD8+ T cells to express exhaustion marker PD-1 at a greater rate (~43%) than pre-exercise blood serum (Dorneles et al., 2020). Mobilization of exhausted T cells was not reported in repeated exercise studies due to their study design (van der Geest et al., 2017; Wong et al., 2021). Acute exercise studies investigating CTLA-4+ exhausted T cells demonstrated unchanged levels in response to exercise, and thus no mobilization (Gustafson et al., 2017; Cury-Boaventura et al., 2018). Unlike senescent CD8+ T cells, the mobilization of exhausted CD8+ T cells may be dependent on the surface protein markers.
Similar to senescent CD8+ T cells, the type and intensity of acute exercise influenced the mobilization of PD-1+ exhausted T cells. High intensity and exhaustive exercise mobilized PD-1+ T cells greater than moderate intensity and endurance exercise, respectively (Gustafson et al., 2017; Wadley et al., 2020). Furthermore, endurance exercise mobilized PD-1+ T cells to a greater extent than resistance exercise (Schenk et al., 2021).
Sedentary Lifestyle May Increase Exhausted CD8+ T Cells
Three studies investigated the effects of repeated exercise on PD-1+ and CTLA-4+ exhausted CD8+ T cells; two indicated that a sedentary lifestyle is linked to an increase in exhausted T cells. Cross-sectional data demonstrated that sedentary adult males, participating in <1 hour of scheduled physical activity per day, had more PD-1+ exhausted T cells than active individuals (Gustafson et al., 2017). After a 6-month horticultural therapy which included walking as an exercise intervention, older adults aged 58–76 years had less CTLA-4+ exhausted T cells than a control group not participating (Wong et al., 2021). Physical activity levels were not measured in the intervention study, thus it cannot be determined if the control group were truly sedentary (Wong et al., 2021). The third study investigating repeated exercise reported an increase in CTLA-4+ exhausted T cells after a 4-day walking event (van der Geest et al., 2017). The older adult participants were physically active (mean physical activity hours/week: males 8.5 ± 8.0, females 5.8 ± 5.9) and likely trained to walk the 30 km each day at the event. Overall, a sedentary lifestyle may lead to an increase in exhausted CD8+ T cells.
Discussion
A systematic search of the literature identified 30 studies that investigated the impact of exercise on protein-surface-marker-defined senescent and/or exhausted CD8+ T cell populations. Only 30% (n = 7) of eligible studies examined exhausted CD8+ T cells, highlighting the lack of relevant studies on this cell type. Overall, it appears that acute exercise can induce mobilization of senescent and exhausted CD8+ T cells. The influence of repeated exercise on limiting senescent/exhausted CD8+ T cells includes: increased cardiorespiratory fitness may protect against the accumulation of senescent CD8+ T cells, endurance resistance training appears to reduce senescent CD8+ T cells in sedentary older adults, and a sedentary lifestyle may lead to an increase in exhausted CD8+ T cells. However, the number of studies available to conceive these findings was limited such that only two studies were designed appropriately to possibly generate the results in each of the three previous findings. More research is required to confirm the influence of repeated exercise on senescent and exhausted CD8+ T cells.
A limited number of studies have investigated the influence of repeated exercise on senescent and exhausted T cells. The shortage of evidence-based investigations on this topic can in part be attributed to the wide variety of terminologies, and inconsistent definitions, for senescent and exhausted T cells. The requirement for senescent and exhausted CD8+ T cell populations to be defined by protein surface markers restricted the inclusion of some studies in this review. The terminology and definitions of exhausted and senescent T cell populations must be clarified and employed systematically in future studies. Additional investigation will be required to determine the clinical relevance of the underlying relationships between repeated exercise and senescent and exhausted T cells. A clearer understanding of these relationships will inform the development of more effective treatment and management strategies for healthy aging, cancer prevention, and vaccine efficiency.
Exercise can mobilize senescent and exhausted CD8+ T cells immediately after an acute bout of physical activity, with levels returning to normal shortly after. It is well-established that exercise elicits transient leukocytosis; however, senescent and exhausted T cells may be preferentially mobilized under certain conditions (Deuster et al., 1988; Turner and Brum, 2017; Ross et al., 2018). Both senescent and exhausted T cells were shown to increase in mobilization with higher intensity exercise (Krüger et al., 2016; Gustafson et al., 2017; LaVoy et al., 2017; Wadley et al., 2020; Schenk et al., 2021). Additionally, older or CMV seropositive individuals demonstrated preferential mobilization of senescent T cells only, likely due to a lack of studies investigating exhausted T cells (Turner et al., 2010; Simpson et al., 2011; Bigley et al., 2012; Lavoy et al., 2014; LaVoy et al., 2017; Ross et al., 2018). Other studies, with differing terminology, demonstrate similar findings such that terminally differentiated lymphocytes exhibiting a senescent phenotype can be selectively mobilized after an acute bout of exercise (Cao Dinh et al., 2016). Unlike PD-1+ exhausted CD8+ T cells, CTLA-4+ exhausted CD8+ T cells were unchanged after acute exercise. A study exposing CD8+ T cells to exercise-induced metabolites supports this outcome (Rundqvist et al., 2020). Further research is required to understand, and confirm, the mobilization of differing exhausted CD8+ T cells in response to acute exercise.
Lymphocytes mobilized by acute exercise are more sensitive to apoptosis through intrinsic and extrinsic apoptotic pathways explained in detail in a review by Simpson (2011). The removal of lymphocytes, and specifically senescent T cells, purportedly creates “immune space” for the production of naïve T cells which are more immunologically responsive. It is yet to be confirmed whether senescent T cells undergo apoptosis at a greater rate than other T cell populations. Senescent T cells can be resistant to some apoptotic pathways, but evidence indicates that exercise-induced apoptotic pathways involve oxidative stress where senescent T cells may be less tolerant than other T cell populations. Even so, if senescent T cells are preferentially mobilized it is likely they are also exposed to apoptotic pathways. Frequent bouts of acute exercise and subsequent T cell shifts may have an accumulative long-term restorative effect on the immune system by “making space” for naïve T cells to increase the TCR repertoire (Simpson, 2011). Therefore, the mobilization of senescent and (some) exhausted T cells may account for the benefits of repeated exercise.
A secondary outcome of this systematic review was that senescent CD8+ T cells may be influenced by sex. Males appear to have a higher proportion of CD28−/CD57+ senescent CD8+ T cells than females (Brown et al., 2014). The influence of sex on the immune response and cells is well-established; however, studies investigating the regulation of senescent T cells are lacking, and outcomes of current studies are inconsistent (Al-Attar et al., 2016). Some studies report that males have greater numbers of senescent T cells than females (Hirokawa et al., 2013; Di Benedetto et al., 2015; Nacka-Aleksić et al., 2020), whereas other studies did not identify substantial differences between men and women (Al-Attar et al., 2016; Reed et al., 2019). One of the studies showing a higher number of senescent T cells in males proposed that the sex hormone estrogen may regulate longevity, thus protecting females from age-related immune decline, such as the loss of surface marker CD28 (Hirokawa et al., 2013). Future studies investigating senescent T cell populations should account for sex as a potential confounder, and employ the appropriate experimental or statistical controls.
It is recognized that mechanisms other than apoptosis may be behind the beneficial effect of repeated exercise limiting senescent and exhausted CD8+ T cell accumulation. Other possible mechanisms are detailed elsewhere and may include exercise-induced delayed thymic atrophy (Duggal et al., 2018) or engagement in repeated exercise reflecting a generally healthier lifestyle including better nutrition (Ravaglia et al., 2000; Wu et al., 2019). Another limitation in the review is the assumption that cardiorespiratory fitness is the sole result of repeated exercise. Cardiorespiratory fitness is a heritable trait and genetic factors determined ~70% of the relationship between exercise and an individual's VO2max score (Mustelin et al., 2011). Future studies will need to determine that those with increased cardiorespiratory fitness and low senescent CD8+ T cells numbers is related to exercise, and not due to genetic factors predisposing the person to delayed immunosenescence, and a high VO2max score. Future work should explore whether a sedentary lifestyle leads to increased accumulation of senescent CD8+ T cells, and evaluate exercise and physical activity interventions that could protect against immunosenescence.
Future Directions
Approaches to reversing exhausted and senescent CD8+ T cell phenotype are emerging, however prevention remains important as accumulation of these cells is linked to decreased vaccine efficiency and increased cancer risk linked to impaired immunosurveillance (Bigley et al., 2013; Pawelec, 2019; Thomas et al., 2020). Exercise has been investigated as an adjunct therapy for improving both vaccination and cancer outcomes (Simpson et al., 2012; Neilson et al., 2014; Campbell and Turner, 2018; Duggal et al., 2019; Hwang et al., 2020). A meta-analysis reported that an active lifestyle involving high levels of leisure activities can reduce all-cause cancer rates by 46% (Bigley et al., 2013). Single bouts as well as habitual exercise before vaccination can improve immune responses, and thus vaccine efficiency (Duggal et al., 2019). However, few studies have examined this relationship with a focus on senescent and/or exhausted CD8+ T cells. The lack of evidence makes it difficult to establish the mechanistic or clinical relevance of regular physical exercise programs for clinical applications and the general population. One possible mechanism details the ability of intense cardiorespiratory exercise to induce an epigenetic mechanism, microRNA expression, to regulate telomere homeostasis in white blood cells to improve immune function and physical health (Chilton et al., 2014). This mechanism would benefit senescent T cells given their known shortened telomeres, as individuals engaging in exercise have longer telomeres (Silva et al., 2016). However, the mechanism was demonstrated in a heterogenous white blood cell population, and future studies will need to isolate senescent CD8+ T cells. This mechanism could be responsible for the ability of exercise to maintain telomere lengths, and consequently improve immune function. As well as identifying the mechanism(s) underlying the benefits of exercise, future studies should determine whether this impact is clinically meaningful, particularly in senescent-prone individuals such as the older adults or cancer patients.
Future work should investigate if epigenetics can provide an insight into the association between latent CMV infection and increased numbers of senescent CD8+ T cells (Simpson et al., 2012). Several of the included studies in this review have detailed the influence of CMV on senescent CD8+ T cells. The chronic stimulation of cytotoxic T cells from CMV infection drives their senescence, and some researchers describe the same model for CMV's association with exhausted cytotoxic T cells (Simpson et al., 2016). We propose an epigenetic mechanism, specifically DNA methylation, that regulates this model. Upon responding to a viral infection, cytotoxic T cells undergo genome-wide DNA methylation remodeling toward an effector phenotype (Schlums et al., 2015). The DNA methylome of CD28− senescent T cells are unique to other T cell phenotypes and contributes to their functional characteristics (Suarez-Álvarez et al., 2016). Therefore, we consider that the chronic stimulation of CMV drives DNA methylation remodeling within cytotoxic T cells, and results in the generation of senescent T cells. A similar theory has been proposed for natural killer (NK) cells, but data are sparse for CD8+ T cells (Schlums et al., 2015). Understanding the molecular mechanism underlying senescent T cell development driven by CMV infection may lead to novel interventions to prevent senescent T cell accumulation. Most importantly, future studies should account for the association between CMV and senescent T cells.
The immunological continuum throughout life needs to be fully characterized to develop personalized and predictive medicine approaches to optimize immune health. The immune risk profile describes a set of immunological parameters that currently predict morbidity and mortality; however, the profile is restricted to immune cell ratios and limited protein biomarkers (Simpson and Guy, 2010). Cutting edge single-cell technologies should provide in-depth profiling of immune cell populations that generate a signature of immune decline over time. Algorithmic frameworks that combine multiparametric lifestyle data and molecular markers are required to develop personalized immune medicine approaches. These frameworks will consist of tests that quantify immune decline. Personalized medicine is already a reality in some areas of healthcare, such as cancer therapy, where lifestyle or pharmacology interventions are tailored to the individual. In the case of declining immune health, these interventions could boost vaccine efficiency or prevent cancer. Personalized and predictive medicine should be accompanied by new clinical/practical guidelines on managing immune health and immunosenescence.
Conclusion
Studies show that repeated exercise can be beneficial in limiting the number of senescent and exhausted CD8+ T cells. Exercise-induced preferential mobilization of senescent CD8+ T cells, and the subsequent promotion of apoptosis in the peripheral blood compartment, is the proposed underlying mechanism. The clinical relevance of the effect of exercise on exhausted and senescent CD8+ T cells has yet to be determined, but with effective clinical and lifestyle implementation could prevent cancer and improve vaccine efficiency. Future studies investigating the effects of exercise should focus on clearly defining the target exhausted and senescent CD8+ T cell populations, and control for confounding variables such as sex and CMV status.
Data Availability Statement
The original contributions presented in the study are included in the article/Supplementary Material, further inquiries can be directed to the corresponding author/s.
Author Contributions
TD conducted the systematic search and drafted the manuscript. TD and DP screened articles. DP, SR, WT, and AB revised and edited the manuscript. All authors contributed to the article and approved the submitted version.
Conflict of Interest
The authors declare that the research was conducted in the absence of any commercial or financial relationships that could be construed as a potential conflict of interest.
Publisher's Note
All claims expressed in this article are solely those of the authors and do not necessarily represent those of their affiliated organizations, or those of the publisher, the editors and the reviewers. Any product that may be evaluated in this article, or claim that may be made by its manufacturer, is not guaranteed or endorsed by the publisher.
Acknowledgments
The authors gratefully thank the University of Canberra Research Institute of Sport and Exercise, and QIMR Berghofer Medical Research Institute for supporting this work.
Supplementary Material
The Supplementary Material for this article can be found online at: https://www.frontiersin.org/articles/10.3389/fphys.2021.668327/full#supplementary-material
Abbreviations
CMV, cytomegalovirus; IRP, immune risk profile; IFN-γ, interferon-γ; IL-6, interleukin-6; NIH, National Institutes of Health; MPO, plasma myeloperoxidase; NK, natural killer; PRISMA, preferred reporting items for systematic reviews and meta-analyses; QA, quality assessment; Tex, exhausted T cell; Ts, senescent T cell; VO2max, maximal aerobic capacity.
References
Al-Attar, A., Presnell, S. R., Peterson, C. A., Thomas, D. T., and Lutz, C. T. (2016). The effect of sex on immune cells in healthy aging: elderly women have more robust natural killer lymphocytes than do elderly men. Mech. Ageing Dev. 156, 25–33. doi: 10.1016/j.mad.2016.04.001
Bastos, M. F., Matias, M. S. T., Alonso, A. C., Silva, L. C. R., de Araújo, A. L., Silva, P. R., et al. (2020). Moderate levels of physical fitness maintain telomere length in non-senescent T CD8+ cells of aged men. Clinics (São Paulo) 75:e1628. doi: 10.6061/clinics/2020/e1628
Berger, S. L., Kouzarides, T., Shiekhattar, R., and Shilatifard, A. (2009). An operational definition of epigenetics. Genes Dev. 23, 781–783. doi: 10.1101/gad.1787609
Bigley, A. B., Lowder, T. W., Spielmann, G., Rector, J. L., Pircher, H., Woods, J. A., et al. (2012). NK-cells have an impaired response to acute exercise and a lower expression of the inhibitory receptors KLRG1 and CD158a in humans with latent cytomegalovirus infection. Brain Behav. Immun. 26, 177–186. doi: 10.1016/j.bbi.2011.09.004
Bigley, A. B., Spielmann, G., LaVoy, E. C., and Simpson, R. J. (2013). Can exercise-related improvements in immunity influence cancer prevention and prognosis in the elderly? Maturitas 76, 51–56. doi: 10.1016/j.maturitas.2013.06.010
Bradshaw, D. I., George, J. D., Hyde, A., LaMonte, M. J., Vehrs, P. R., Hager, R. L., et al. (2005). An accurate VO2max nonexercise regression model for 18–65-year-old adults. Res. Q. Exerc. Sport 76, 426–432. doi: 10.1080/02701367.2005.10599315
Brown, F. F., Bigley, A. B., Ross, J. C., LaVoy, E. C., Simpson, R. J., and Galloway, S. D. R. (2015). T-lymphocyte populations following a period of high volume training in female soccer players. Physiol. Behav. 152, 175–181. doi: 10.1016/j.physbeh.2015.09.027
Brown, F. F., Bigley, A. B., Sherry, C., Neal, C. M., Witard, O. C., Simpson, R. J., et al. (2014). Training status and sex influence on senescent T-lymphocyte redistribution in response to acute maximal exercise. Brain Behav. Immun. 39, 152–159. doi: 10.1016/j.bbi.2013.10.031
Campbell, J. P., and Turner, J. E. (2018). Debunking the myth of exercise-induced immune suppression: redefining the impact of exercise on immunological health across the lifespan. Front. Immunol. 9, 648. doi: 10.3389/fimmu.2018.00648
Cao Dinh, H., Bautmans, I., Beyer, I., Onyema, O. O., Liberman, K., De Dobbeleer, L., et al. (2019). Six weeks of strength endurance training decreases circulating senescence-prone T-lymphocytes in cytomegalovirus seropositive but not seronegative older women. Immun. Ageing 16:17. doi: 10.1186/s12979-019-0157-8
Cao Dinh, H., Beyer, I., Mets, T., Onyema, O. O., Njemini, R., Renmans, W., et al. (2016). Effects of physical exercise on markers of cellular immunosenescence: a systematic review. Calcif. Tissue Int. 100, 193–215. doi: 10.1007/s00223-016-0212-9
Chilton, W. L., Marques, F. Z., West, J., Kannourakis, G., Berzins, S. P., O'Brien, B. J., et al. (2014). Acute exercise leads to regulation of telomere-associated genes and microRNA expression in immune cells. PLoS One 9:e92088. doi: 10.1371/journal.pone.0092088
Cosgrove, C., Galloway, S. D., Neal, C., Hunter, A. M., McFarlin, B. K., Spielmann, G., et al. (2012). The impact of 6-month training preparation for an Ironman triathlon on the proportions of naïve, memory and senescent T cells in resting blood. Eur. J. Appl. Physiol. 112, 2989–2998. doi: 10.1007/s00421-011-2273-9
Crespo, J., Sun, H., Welling, T. H., Tian, Z., and Zou, W. (2013). T cell anergy, exhaustion, senescence, and stemness in the tumor microenvironment. Curr. Opin. Immunol. 25, 214–221. doi: 10.1016/j.coi.2012.12.003
Curran, M., Campbell, J., Drayson, M., Andrews, R., and Narendran, P. (2019). Type 1 diabetes impairs the mobilisation of highly-differentiated CD8+T cells during a single bout of acute exercise. Exerc. Immunol. Rev. 25, 64–82.
Cury-Boaventura, M. F., Gorjão, R., de Moura, N. R., Santos, V. C., Bortolon, J. R., Murata, G. M., et al. (2018). The effect of a competitive futsal match on T lymphocyte surface receptor signaling and functions. Front. Physiol. 9:202. doi: 10.3389/fphys.2018.00202
Dan, L., Liu, L., Sun, Y., Song, J., Yin, Q., Zhang, G., et al. (2020). The phosphatase PAC1 acts as a T cell suppressor and attenuates host antitumor immunity. Nat. Immunol. 21, 287–297. doi: 10.1038/s41590-019-0577-9
Deuster, P. A., Curiale, A. M., Cowan, M. L., and Finkelman, F. D. (1988). Exercise-induced changes in populations of peripheral blood mononuclear cells. Med. Sci. Sports Exerc. 20, 276–280. doi: 10.1249/00005768-198806000-00011
Di Benedetto, S., Derhovanessian, E., Steinhagen-Thiessen, E., Goldeck, D., Muller, L., and Pawelec, G. (2015). Impact of age, sex and CMV-infection on peripheral T cell phenotypes: results from the Berlin BASE-II Study. Biogerontology 16, 631–643. doi: 10.1007/s10522-015-9563-2
Dorneles, G. P., da Silva, I. M., Santos, M. A., Elsner, V. R., Fonseca, S. G., Peres, A., et al. (2020). Immunoregulation induced by autologous serum collected after acute exercise in obese men: a randomized cross-over trial. Sci. Rep. 10:21735. doi: 10.1038/s41598-020-78750-z
Duggal, N. A., Niemiro, G., Harridge, S. D. R., Simpson, R. J., and Lord, J. M. (2019). Can physical activity ameliorate immunosenescence and thereby reduce age-related multi-morbidity? Nat. Rev. Immunol. 19, 563–572. doi: 10.1038/s41577-019-0177-9
Duggal, N. A., Pollock, R. D., Lazarus, N. R., Harridge, S., and Lord, J. M. (2018). Major features of immunesenescence, including reduced thymic output, are ameliorated by high levels of physical activity in adulthood. Aging Cell 17:e12750. doi: 10.1111/acel.12750
Gustafson, M. P., DiCostanzo, A. C., Wheatley, C. M., Kim, C. H., Bornschlegl, S., Gastineau, D. A., et al. (2017). A systems biology approach to investigating the influence of exercise and fitness on the composition of leukocytes in peripheral blood. J Immunother Cancer 5:30. doi: 10.1186/s40425-017-0231-8
Hirokawa, K., Utsuyama, M., Hayashi, Y., Kitagawa, M., Makinodan, T., and Fulop, T. (2013). Slower immune system aging in women versus men in the Japanese population. Immun. Ageing 10:19. doi: 10.1186/1742-4933-10-19
Huff, W. X., Kwon, J. H., Henriquez, M., Fetcko, K., and Dey, M. (2019). The evolving role of CD8(+)CD28(-) immunosenescent T cells in cancer immunology. Int. J. Mol. Sci. 20:2810. doi: 10.3390/ijms20112810
Hwang, J. H., McGovern, J., Minett, G. M., Della Gatta, P. A., Roberts, L., Harris, J.M., et al. (2020). Mobilizing serum factors and immune cells through exercise to counteract age-related changes in cancer risk. Exerc. Immunol. Rev. 26, 80–99.
Ingram, L. A., Simpson, R. J., Malone, E., and Florida-James, G. D. (2015). Sleep disruption and its effect on lymphocyte redeployment following an acute bout of exercise. Brain Behav. Immun. 47, 100–108. doi: 10.1016/j.bbi.2014.12.018
Karim, A. A. P., and Jabbar, B. P. (2018). Effects of hypobaric endurance training on graded exercise induced lymphocyte mobilization, senescence and their surface thiol levels in elite male athletes. Int. J. Appl. Exer. Physiol. 7, 48–55. doi: 10.22631/ijaep.v7i1.227
Krüger, K., Alack, K., Ringseis, R., Mink, L., Pfeifer, E., Schinle, M., et al. (2016). Apoptosis of T-cell subsets after acute high-intensity interval exercise. Med. Sci. Sports Exerc. 48, 2021–2029. doi: 10.1249/mss.0000000000000979
Lavoy, E. C., Bigley, A. B., Spielmann, G., Rector, J. L., Morrison, M. R., O'Connor, D. P., et al. (2014). CMV amplifies T-cell redeployment to acute exercise independently of HSV-1 serostatus. Med. Sci. Sports Exerc. 46, 257–267. doi: 10.1249/MSS.0b013e3182a5a0fb
LaVoy, E. C., Hussain, M., Reed, J., Kunz, H., Pistillo, M., Bigley, A. B., et al. (2017). T-cell redeployment and intracellular cytokine expression following exercise: effects of exercise intensity and cytomegalovirus infection. Physiol. Rep. 5:e13070. doi: 10.14814/phy2.13070
Ling, C., and Ronn, T. (2014). Epigenetic adaptation to regular exercise in humans. Drug Discov. Today 19, 1015–1018. doi: 10.1016/j.drudis.2014.03.006
McElhaney, J. E., Kuchel, G. A., Zhou, X., Swain, S. L., and Haynes, L. (2016). T-cell immunity to influenza in older adults: a pathophysiological framework for development of more effective vaccines. Front. Immunol. 7:41. doi: 10.3389/fimmu.2016.00041
Minuzzi, L. G., Rama, L., Chupel, M. U., Rosado, F., Dos Santos, J. V., Simpson, R., et al. (2018). Effects of lifelong training on senescence and mobilization of T lymphocytes in response to acute exercise. Exerc. Immunol. Rev. 24, 72–84.
Moher, D., Liberati, A., Tetzlaff, J., Altman, D. G., and Group, P. (2009). Preferred reporting items for systematic reviews and meta-analyses: the PRISMA statement. PLoS Med. 6:e1000097. doi: 10.1371/journal.pmed.1000097
Mustelin, L., Latvala, A., Pietiläinen, K. H., Piirilä, P., Sovijärvi, A. R., Kujala, U. M., et al. (2011). Associations between sports participation, cardiorespiratory fitness, and adiposity in young adult twins. J. Appl. Physiol. 110, 681–686. doi: 10.1152/japplphysiol.00753.2010
Nacka-Aleksić, M., Stojić-Vukanić, Z., Pilipović, I., Blagojević, V., Kotur-Stevuljević, J., and Leposavić, G. (2020). Sex as a confounding factor in the effects of ageing on rat lymph node t cell compartment. Exp. Gerontol. 142:111140. doi: 10.1016/j.exger.2020.111140
National Heart Lung Blood Institute. (2021). Study Quality Assessment Tools. National Institutes of Health (NIH). Available online at: https://www.nhlbi.nih.gov/health-topics/study-quality-assessment-tools (accessed January 1, 2021).
Neilson, H. K., Conroy, S. M., and Friedenreich, C. M. (2014). The influence of energetic factors on biomarkers of postmenopausal breast cancer risk. Curr. Nutr. Rep. 3, 22–34. doi: 10.1007/s13668-013-0069-8
Pawelec, G. (2019). Is there a positive side to T cell exhaustion? Front. Immunol. 10:111. doi: 10.3389/fimmu.2019.00111
Pedersen, B. K., and Saltin, B. (2015). Exercise as medicine - evidence for prescribing exercise as therapy in 26 different chronic diseases. Scand. J. Med. Sci. Sports 25(Suppl 3), 1–72. doi: 10.1111/sms.12581
Ravaglia, G., Forti, P., Maioli, F., Bastagli, L., Facchini, A., Mariani, E., et al. (2000). Effect of micronutrient status on natural killer cell immune function in healthy free-living subjects aged ≥90 years. Am. J. Clin. Nutr. 71, 590–598. doi: 10.1093/ajcn/71.2.590
Reed, R. G., Al-Attar, A., Presnell, S. R., Lutz, C. T., and Segerstrom, S. C. (2019). A longitudinal study of the stability, variability, and interdependencies among late-differentiated T and NK cell subsets in older adults. Exp. Gerontol. 121, 46–54. doi: 10.1016/j.exger.2019.03.006
Rogers, M. A., Hagberg, J. M., Martin, W. H. III., Ehsani, A. A., and Holloszy, J. O. (1990). Decline in VO2max with aging in master athletes and sedentary men. J. Appl. Physiol. 68, 2195–2199. doi: 10.1152/jappl.1990.68.5.2195
Ross, M., Ingram, L., Taylor, G., Malone, E., Simpson, R. J., West, D., et al. (2018). Older men display elevated levels of senescence-associated exercise-responsive CD28(null) angiogenic T cells compared with younger men. Physiol. Rep. 6:e13697. doi: 10.14814/phy2.13697
Rundqvist, H., Veliça, P., Barbieri, L., Gameiro, P. A., Bargiela, D., Gojkovic, M., et al. (2020). Cytotoxic T-cells mediate exercise-induced reductions in tumor growth. Elife 9:e59996. doi: 10.7554/eLife.59996
Schenk, A., Joisten, N., Walzik, D., Koliamitra, C., Schoser, D., Bloch, W., et al. (2021). Acute exercise impacts AhR and PD-1 levels of CD8(+) T-cells-Exploratory results from a randomized cross-over trial comparing endurance versus resistance exercise. Eur. J. Appl. Physiol. 121, 637–644. doi: 10.1007/s00421-020-04552-w
Schlums, H., Cichocki, F., Tesi, B., Theorell, J., Beziat, V., Holmes, T. D., et al. (2015). Cytomegalovirus infection drives adaptive epigenetic diversification of NK cells with altered signaling and effector function. Immunity 42, 443–456. doi: 10.1016/j.immuni.2015.02.008
Shimizu, K., Suzuki, N., Imai, T., Aizawa, K., Nanba, H., Hanaoka, Y., et al. (2011). Monocyte and T-cell responses to exercise training in elderly subjects. J. Strength Cond. Res. 25, 2565–2572. doi: 10.1519/JSC.0b013e3181fc5e67
Silva, L. C., de Araújo, A. L., Fernandes, J. R., Matias Mde, S., Silva, P. R., Duarte, A. J., et al. (2016). Moderate and intense exercise lifestyles attenuate the effects of aging on telomere length and the survival and composition of T cell subpopulations. Age (Dordr). 38:24. doi: 10.1007/s11357-016-9879-0
Simpson, R., Spielmann, G., Bigley, A., Rector, J., Morrison, M., La Voy, E., et al. (2011). Latent CMV and EBV infections and the mobilization of viral-specific and senescent T-cells with exercise. J. Immunol. 186(1 Suppl.):102.6.
Simpson, R. J. (2011). Aging, persistent viral infections, and immunosenescence: can exercise “make space”? Exerc. Sport Sci. Rev. 39, 23–33. doi: 10.1097/JES.0b013e318201f39d
Simpson, R. J., Bigley, A. B., Spielmann, G., LaVoy, E. C., Kunz, H., and Bollard, C. M. (2016). Human cytomegalovirus infection and the immune response to exercise. Exerc. Immunol. Rev. 22, 8–27.
Simpson, R. J., Cosgrove, C., Chee, M. M., McFarlin, B. K., Bartlett, D. B., Spielmann, G., et al. (2010). Senescent phenotypes and telomere lengths of peripheral blood T-cells mobilized by acute exercise in humans. Exerc. Immunol. Rev. 16, 40–55.
Simpson, R. J., and Guy, K. (2010). Coupling aging immunity with a sedentary lifestyle: has the damage already been done?–a mini-review. Gerontology 56, 449–458. doi: 10.1159/000270905
Simpson, R. J., Lowder, T. W., Spielmann, G., Bigley, A. B., LaVoy, E. C., and Kunz, H. (2012). Exercise and the aging immune system. Ageing Res. Rev. 11, 404–420. doi: 10.1016/j.arr.2012.03.003
Spielmann, G., Bollard, C. M., Bigley, A. B., Hanley, P. J., Blaney, J. W., LaVoy, E. C., et al. (2014). The effects of age and latent cytomegalovirus infection on the redeployment of CD8+ T cell subsets in response to acute exercise in humans. Brain Behav. Immun. 39, 142–151. doi: 10.1016/j.bbi.2013.05.003
Spielmann, G., McFarlin, B. K., O'Connor, D. P., Smith, P. J., Pircher, H., and Simpson, R. J. (2011). Aerobic fitness is associated with lower proportions of senescent blood T-cells in man. Brain Behav. Immun. 25, 1521–1529. doi: 10.1016/j.bbi.2011.07.226
Suarez-Álvarez, B., Rodríguez, R. M., Schlangen, K., Raneros, A. B., Márquez-Kisinousky, L., Fernández, A. F., et al. (2016). Phenotypic characteristics of aged CD4(+) CD28(null) T lymphocytes are determined by changes in the whole-genome DNA methylation pattern. Aging Cell 16, 293–303. doi: 10.1111/acel.12552
Theall, B., Wang, H., Kuremsky, C. A., Cho, E., Hardin, K., Robelot, L., et al. (2020). Allostatic stress load and CMV serostatus impact immune response to maximal exercise in collegiate swimmers. J. Appl. Physiol. (1985). 128, 178–188. doi: 10.1152/japplphysiol.00646.2019
Thomas, R., Wang, W., and Su, D. M. (2020). Contributions of age-related thymic involution to immunosenescence and inflammaging. Immun. Ageing 17:2. doi: 10.1186/s12979-020-0173-8
Turner, J. E. (2016). Is immunosenescence influenced by our lifetime “dose” of exercise? Biogerontology 17, 581–602. doi: 10.1007/s10522-016-9642-z
Turner, J. E., Aldred, S., Witard, O. C., Drayson, M. T., Moss, P. M., and Bosch, J. A. (2010). Latent Cytomegalovirus infection amplifies CD8 T-lymphocyte mobilisation and egress in response to exercise. Brain Behav. Immun. 24, 1362–1370. doi: 10.1016/j.bbi.2010.07.239
Turner, J. E., and Brum, P. C. (2017). Does regular exercise counter T cell immunosenescence reducing the risk of developing cancer and promoting successful treatment of malignancies? Oxid. Med. Cell. Longev. 2017:4234765. doi: 10.1155/2017/4234765
van der Geest, K. S. M., Wang, Q., Eijsvogels, T. M. H., Koenen, H. J. P., Joosten, I., Brouwer, E., et al. (2017). Changes in peripheral immune cell numbers and functions in octogenarian walkers - an acute exercise study. Immun. Ageing 14:5. doi: 10.1186/s12979-017-0087-2
Wadley, A. J., Cullen, T., Vautrinot, J., Keane, G., Bishop, N. C., and Coles, S. J. (2020). High intensity interval exercise increases the frequency of peripheral PD-1+ CD8(+) central memory T-cells and soluble PD-L1 in humans. Brain Behav. Immun. Health 3:100049. doi: 10.1016/j.bbih.2020.100049
Wang, J. S., Chen, W. L., and Weng, T. P. (2011). Hypoxic exercise training reduces senescent T-lymphocyte subsets in blood. Brain Behav. Immun. 25, 270–278. doi: 10.1016/j.bbi.2010.09.018
Wong, G. C. L., Ng, T. K. S., Lee, J. L., Lim, P. Y., Chua, S. K. J., Tan, C., et al. (2021). Horticultural therapy reduces biomarkers of immunosenescence and inflammaging in community-dwelling older adults: a feasibility pilot randomized controlled trial. J. Gerontol. A Biol. Sci. Med. Sci. 76, 307–317. doi: 10.1093/gerona/glaa271
Keywords: exhausted, senescent, T cells, immune cells, exercise, fitness
Citation: Donovan T, Bain AL, Tu W, Pyne DB and Rao S (2021) Influence of Exercise on Exhausted and Senescent T Cells: A Systematic Review. Front. Physiol. 12:668327. doi: 10.3389/fphys.2021.668327
Received: 25 February 2021; Accepted: 29 July 2021;
Published: 20 August 2021.
Edited by:
James Edward Turner, University of Bath, United KingdomReviewed by:
Mark Daniel Ross, Edinburgh Napier University, United KingdomEmily C. LaVoy, University of Houston, United States
Copyright © 2021 Donovan, Bain, Tu, Pyne and Rao. This is an open-access article distributed under the terms of the Creative Commons Attribution License (CC BY). The use, distribution or reproduction in other forums is permitted, provided the original author(s) and the copyright owner(s) are credited and that the original publication in this journal is cited, in accordance with accepted academic practice. No use, distribution or reproduction is permitted which does not comply with these terms.
*Correspondence: Sudha Rao, sudha.rao@qimrberghofer.edu.au
†These authors have contributed equally to this work and share last authorship